Oxygen is a highly reactive gas that is odourless, tasteless and transparent and makes up just under 21% of atmospheric air (Lister et al, 2020). It is required for cellular respiration and once inhaled into the lungs is distributed around the body via the circulatory system, where it becomes part of the energy-making process within the cells (Waugh and Grant, 2018). Oxygen is therefore vital for life because reduced levels of oxygen in the blood (hypoxaemia) or lack of oxygen at a cellular level (hypoxia—PaO2 below 8 kPa (60 mmHg)) can lead to a number of serious complication and can be potentially fatal (Schlag and Redl, 2012; Lister et al, 2020). Oxygen therapy, also referred to as supplementary oxygen, may be required for patients who need treatment for, or are at risk of, hypoxaemia, thereby preventing the occurrence of a hypoxic injury (O'Driscoll et al, 2017). However, although the administration of oxygen will improve the oxygenation of the patient, it does not treat the initial cause of hypoxaemia, which should be investigated as a matter of urgency.
Oxygen saturation levels (SpO2) are used to monitor the level of haemoglobin carrying oxygen in the blood, relative to the amount of haemoglobin that is not carrying oxygen. These levels can be obtained with the use of a pulse oximetry device, which can be attached to a patient's finger, toe, earlobe or nose to retrieve an accurate reading (O'Driscoll et al, 2017). The monitor displays a reading of how well saturated the patient's haemoglobin is with oxygen, and this number is presented as a percentage. Target saturations for a healthy individual should be in the range 95-100% (indicating that the haemoglobin in the blood is almost fully saturated with oxygen). If a SpO2 reading is under 95%, this could indicate early stages of hypoxaemia; however, lower SpO2 readings can be expected in patients with chronic lung disease, such as chronic obstructive pulmonary disease (COPD), chronic bronchitis and emphysema. For patients suffering from severe COPD, it would not be unusual to see baseline oxygen saturation of between 88% and 92% (O'Driscoll et al, 2017).
It is important to recognise that any patient, in any environment, at any time, may need oxygen therapy and it is one of the first-line strategies for acutely ill patients; consequently, in all practice settings, health professionals must understand the risks and guidance on oxygen delivery systems and administration protocols (Moore, 2017). This is even more imperative when faced with pandemics, such as COVID-19, as guidelines may need to be amended and symptom management adaptations may be required depending on how physiological functioning is affected. Box 1 provides a list of the adaptations that are necessary when administering oxygen therapy to patients who have tested positive for COVID-19 and have moderate symptoms.
Oxygen dispensing and documentation
Oxygen must be regarded as a drug and as such should be prescribed (Joint Formulary Committee (JFC), 2020). However, in some cases, such as life-threatening situations, oxygen can be given and adjusted before an individual prescription has been written (O'Driscoll, 2017). Prescriptions should include the percentage of oxygen to be delivered (or the flow rate), the duration of the oxygen therapy and the target oxygen saturation levels for the individual patient (see Table 1). Patients not achieving the target saturation level or experiencing a rapid decline in saturation levels (reduced by 3%) must be reviewed, so that possible causes of deterioration can be identified and appropriate investigations and treatments commenced (O'Driscoll, 2017). This could include an increase or decrease in the percentage of oxygen to be delivered and associated flow rates, which must be made and documented by qualified staff who have undertaken appropriate training in titrating the amount of oxygen delivered according to the patient's oxygen saturation levels (O'Driscoll, 2017). It is also worth noting that in some cases low levels may be recorded due to equipment failure and therefore it is imperative that equipment is also regularly checked to ensure it is working correctly ie at each medication round and when there is a change in saturation levels.
% SPO2 | Examples of when required |
---|---|
88–92% | For patients with chronic obstructive pulmonary disease (COPD) or who are at risk of hypercapnic respiratory failure for other reasons, eg morbid obesity, neuromuscular disorders, chest wall deformities |
94–98% | For acutely ill patients not at risk of hypercapnic respiratory failure |
Monitoring of patients receiving oxygen therapy
As with every administered drug or treatment, the effects of oxygen therapy must be regularly reviewed and monitored, as several serious complications (see Box 2) are associated with the administration of oxygen (JFC, 2020). As oxygen cannot be stored in the body, giving too much is unnecessary and some patients (particularly those with respiratory and cardiovascular disorders) may be harmed by high levels of oxygen as this can lead to increased levels of carbon dioxide (O'Driscoll, 2017). Consequently, O'Driscoll et al (2017) advocate that patients' oxygen saturation should be measured:
It also advises that oxygen saturation levels should be monitored for at least 5 minutes:
Oxygen supply
Within clinical settings, if the patient is stationary (ie in bed or a chair) oxygen may be piped from a central supply to the bed area. Similarly, within the operating department, oxygen required for the maintenance of anaesthesia will also come from this central supply. To access oxygen for administration from the central supply, recognition of the correct gas outlet is of vital importance. Once identified, these outlets can be used to connect either a pipeline to link to a ventilator or anaesthetic machine or an oxygen flowmeter (Woodhead and Fudge, 2012).
There are various types of wall outlets available in order to provide different types of medical gas, since as well as oxygen, nitrous oxide, medical air and a vacuum outlet for suction are commonly used in clinical settings. A ‘tug test’ should be completed to confirm the insertion of each pipeline or flowmeter into its appropriate outlet (Woodhead and Fudge, 2012). As a key safety feature, the outlets and pipeline connectors or flowmeter connectors are designed so that it is impossible to insert the wrong connector into the outlet (Healthcare Safety Investigation Branch, 2019). However, if a flowmeter is inserted into the wall outlet and is not checked by the practitioner, it would be possible to deliver the wrong gas to the patient because all flowmeters have a ‘fir tree’ connection. It has been suggested by NHS Improvement (2016) that caps should be used to cover the medical air outlet in environments where it is unlikely that this gas will be used and if possible these outlets should be removed to minimise the risk of any never events occurring with medical gases.
If a patient is being transported around the hospital, in a community setting or during a patient transfer, oxygen will be administered from an oxygen cylinder. When using an oxygen cylinder, there are several safety features to be aware of as well as a good understanding of the operating instructions (Box 3). It is important to always check the product information on the cylinder to confirm that it is oxygen and not another medical gas, and the label should be checked to ensure that the oxygen is within its use-by date. Oxygen cylinders must also be stored in an area that is clean, dry and well ventilated (Health and Safety Executive (HSE), 2013) and stored vertically, either chained or clamped into place to prevent them from falling (Association of Anaesthetists of Great Britain and Ireland, 2012). It is important not to keep these cylinders in areas where there are flammable materials.
The Fire Triangle (Figure 1) depicts that three components are needed for combustion: fuel, heat and oxygen. The removal of potential fuels and sources of heat minimises the risk of a fire in the clinical environment. Therefore, smoking and naked lights must not be allowed near oxygen cylinders or piped oxygen (HSE, 2013). The Medicines and Healthcare products Regulatory Agency (2013) has warned that there is an increased risk of a serious fire if substances such as dirt, oil, grease or hand creams contaminate connections between medical devices and medical gas cylinders; cleanliness must, therefore, be maintained. This includes ensuring hands are clean, and if alcohol gel has been used, making sure it is completely dry before cylinders are handled due to the flammable nature of the gel.
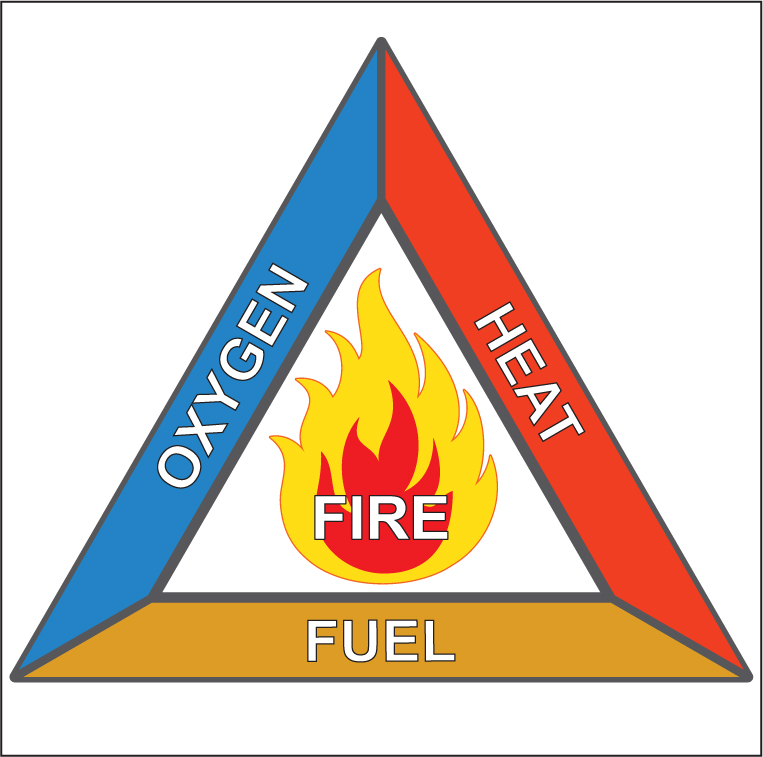
Types of masks and cannulae
There are a plethora of devices that can be used to deliver oxygen to patients, ranging from those that enable the delivery of high percentages of oxygen to devices that facilitate lower and medium levels of oxygen concentration. The exact percentages and associated flow rates for these devices may vary slightly between manufacturers, therefore it is important to work within manufacturers guidelines. For many of the devices, the actual percentage of oxygen delivered will also be variable depending on the flow rate that is set, how well the device conforms to the patient's face and the depth and rate of the patient's respirations (ie fast and shallow breathing often result in lower levels whereas deeper and longer breathing may influence higher oxygen concentration levels) (Moore, 2017). These devices are therefore referred to as variable performance devices.
Variable performance devices
High-concentration reservoir masks, non-rebreathing masks
These masks enable the delivery of high percentages of oxygen to critically ill patients (Resuscitation Council UK, 2015b). As the name suggests, this type of mask is designed to minimise the rebreathing of the patient's expired carbon dioxide, which is facilitated by the use of valves and a reservoir bag (Lister et al, 2020). It is estimated that 60-90% oxygen concentration levels can be delivered when the flow rate is set at 15 litres/minute (O'Driscoll, 2017). However, in order for these masks to be effective, it is important to check the functioning of the values and bag prior to use and apply using the correct technique (Moore, 2017) (Figure 2).
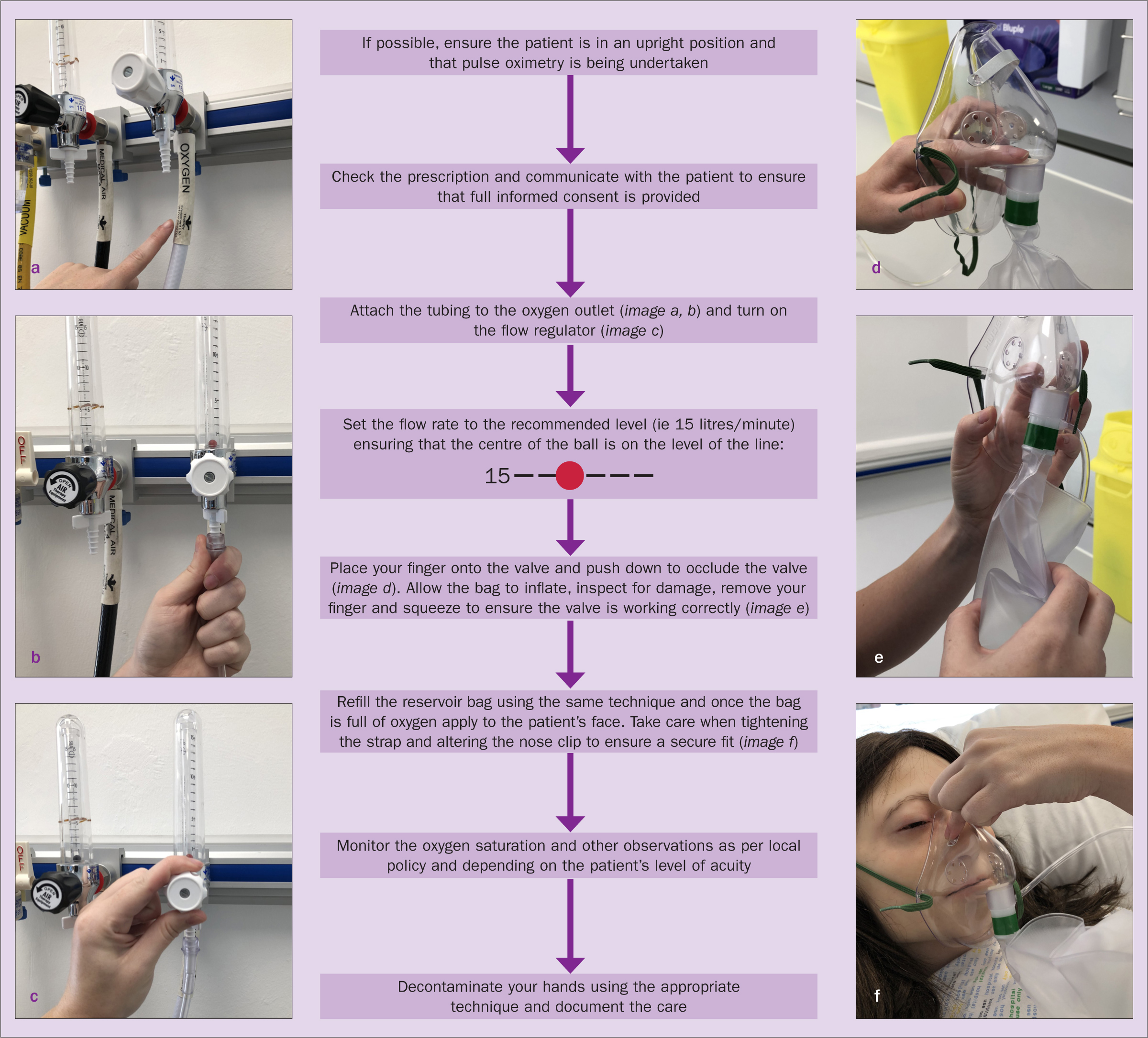
Nasal cannulae
These are used to deliver low to medium concentrations of oxygen (24% to approximately 50%) with flow rates of between 1 and 6 litres/minute; however, flow rates are often restricted to 1-4 litres/minute as nasal dryness and discomfort can be experienced with flow rates above 4 litres/minute (O'Driscoll, 2017) Nasal cannulae have a number of advantages over simple face masks, including being less claustrophobic and easier for patients to use when eating, drinking and conversing (Moore, 2017). However, they cannot be used if patients have nasal obstructions, severe nasal congestion or if the patient is breathing via the mouth only. They can also cause nasal discomfort and may cause pressure damage if not correctly fitted (O'Driscoll, 2017). To reduce nasal discomfort, they are available with an assortment of nasal probe sizes and shapes, which help direct and diffuse the oxygen flow (Lister et al, 2020).
Simple face masks
These masks are designed to deliver low to medium concentrations of oxygen (approximately 40-60%). To achieve this the flow rates should be adjusted between 5 and 10 litres/minute (this may vary slightly between manufacturers). Oxygen is piped into the mask and mixes with the room air entering via the ventilation holes in the side of the mask (these also allow released gases to flow out during exhalation) (Moore, 2017). If this mask is used to deliver oxygen at low flow rates of less than 5 litres/minute, issues may occur with increased resistance to breathing or the re-breathing of exhaled carbon dioxide which can build up inside the mask (O'Driscoll, 2017). Within clinical practice a nasal cannula is often preferred over simple face masks, due to these safety concerns but also comfort and less inspiratory resistance (O'Driscoll, 2017).
Fixed performance masks
Unlike the previous devices, Venturi masks are used to deliver fixed concentrations of oxygen from 24% to 60% via a Venturi entrainer, which uses kinetic energy (Moore, 2017). The percentage of oxygen is fixed regardless of the flow rate being set, as it is the shape of the entrainer and the process of physics that combines oxygen and room air that determines the fixed oxygen concentration that flows into the mask (Table 2). Because they allow very accurate oxygen delivery, these are often used for patients at risk of hypercapnia (O'Driscoll, 2017). For patients with COPD, either a 24% or 28% Venturi mask should be used initially pending the results of blood gas analysis in an emergency (JFC, 2020).
Oxygen flow rate L/minute | Venturi valves | |||||
---|---|---|---|---|---|---|
24 | 28 | 31 | 35 | 40 | 60 | |
20 | 84 | 40 | ||||
15 | 84 | 82 | 30 | |||
12 | 67 | 50 | 24 | |||
10 | 8 | 56 | 41 | |||
8 | 89 | 63 | 46 | |||
6 | 67 | 47 | ||||
4 | 102 | 44 | ||||
2 | 51 |
Humidified oxygen
Normal breathing involves the inhalation of air into the upper respiratory passages, which filter, warm and add moisture to enhance gaseous exchange. If the upper airway passages are bypassed or if these processes are dysfunctional, humidification is often used within clinical practice (O'Driscoll, 2017). Humidification is the process of introducing water vapour, moisture or humidity to the air (Wilkes, 2011). When oxygen therapy is being administered it can cause drying of the respiratory mucosal membranes, which can increase patient discomfort as well as causing further airway damage if the patient receives prolonged exposure to high-flow oxygen without humidification. As such, O'Driscoll et al (2017) suggest that although it is not necessary to humidify oxygen in all cases, humidification is recommended for patients who experience any discomfort associated with dry upper respiratory tract mucosa; patients who have been receiving oxygen via a Venturi mask for more than 24 hours; and individuals who have a tracheostomy in situ.
A number of humidification systems are available to help prevent the damage to the patient's airway. These vary in their level of sophistication but primarily have the same function, that they add molecular water to gas. It is important with all oxygen equipment, including humidifiers, that policies regarding their use are followed to reduce the risk of infection occurring. La Fauci et al (2017) demonstrated that high levels of microbial contamination were present in samples taken from reusable humidification bottles; they concluded that these devices could be involved in the transmission of pathogens. Other devices, which are single patient use, can be used to humidify the medical gases. Heat and moisture exchange (HME) filters can be attached to breathing systems to help prevent damage to the airway as well as the filter providing protection against microbes (Wilkes, 2011). The HME collects the moisture from the expired humid air and holds the water molecules on the filter. Then during inspiration, the water molecules are collected by the gas and the patient breathes in the humid air.
Monitoring end-tidal CO2 levels
End-tidal CO2 (EtCO2) is the level of carbon dioxide that is released at the end of an expired breath. Capnography is used to measure this and the normal range for EtCO2 is considered to be 35-45 mmHg (Woodhead and Fudge, 2012). Monitoring this figure can help to identify success or failure while performing an airway management procedure, for example, the correct placement of an endotracheal tube (ETT). Once familiar with the waveform an EtCO2 produces, an expert will be able to identify a leaking cuff, or if the ETT has been placed too far into the patient and is in the right main bronchus (Dudley et al, 2015). Capnography can also be used to identify a number of physiological functions, for example, it gives an indication of cardiac output and pulmonary blood flow.
There are two types of capnograph, both of which are non-invasive devices. A mainstream capnograph is positioned in between the ETT and the breathing circuit for the patient. In contrast, when using sidestream technology, a long sampling tube will carry the expired air to an infra-red sensor (usually found by the monitoring screen). The transport of gases to the infrared measuring device results in a delay of 1–4 seconds in carbon dioxide measurement and display of the results (Kodali, 2013). Some oxygen masks and nasal cannulae used now also include additional tubing that can be attached to a capnograph to monitor EtCO2, in addition to delivering oxygen. Although the reliability of these readings varies, this can be mediated by the correct use of the capnography equipment (Kasuya et al, 2009).
Capnography monitoring is most often used in the operating department and critical care environments; however, it is now being increasingly used in other departments for specific situations:
Conclusion
The use of oxygen therapy as a treatment strategy is commonplace and for many patients a lifesaving intervention; however, it must be used carefully. This article has not only provided the reader with knowledge to help them appreciate the benefits of oxygen administration but also highlighted the potential risks involved in the application of oxygen to a patient and how following guidelines and adhering to guidelines around prescriptions, environment and the equipment is crucial to the success of oxygen therapy.